David Green
Chicago, Illinois, United States
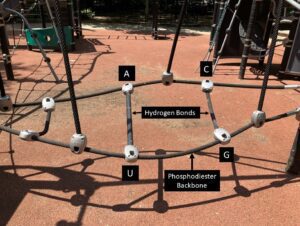
A=adenine, C=cytosine, U=uracil, G=guanine
As an undergraduate at the University of Pennsylvania in the 1950s, I attended a lecture by Harold C. Urey, a Nobel laureate. The subject of his lecture was the origin of life, and he described an experiment that he and his graduate student, Stanley Miller, had performed at the University of Chicago. To determine whether the conditions on prebiotic Earth were conducive for the formation of complex organic compounds, Urey and Miller filled a flask with methane, ammonia, hydrogen, and water vapor to simulate the early atmosphere. Then they sent a continuous electrical spark through the mixture to mimic lightning and analyzed the contents of the flask using paper chromatography.
The chromatogram revealed the presence of five amino acids: glycine, α- and β-alanine, aspartic acid, and α-aminobutyric acid. Because amino acids are the building blocks of proteins, the observations were hailed as consistent with the hypothesis that life could have begun in the primordial soup present on Earth four billion years ago. Recently, the contents of vials remaining from these and other experiments conducted by Miller were examined by high performance liquid chromatography and mass spectrometry. Twenty-two amino acids as well as other organic molecules were identified.1
The experiments of Urey and Miller used a parsimonious concept of Earth’s early atmosphere, limited to just a few gases. However, the primeval soup also contained iron salts and carbonates, substances that could have stabilized the nascent amino acids. In addition, water vapor, carbon dioxide, sulfur dioxide, and to a lesser extent, hydrogen sulfide, carbon monoxide, hydrogen chloride, and hydrogen fluoride were spewing out of erupting volcanos. The sulfurous gases might have contributed to the formation of the sulfur-containing amino acids cysteine and methionine that are incorporated into proteins.
Amino acids are the building blocks of proteins and are linked together in long chains called peptides. Peptide bond formation rarely occurs in an aqueous environment because a water molecule must be eliminated for every peptide bond synthesized. However, Griffith and Vaida2 recently observed the presence of oligomeric peptides at the surface of solutions containing amino acid esters and copper ions. These bonds formed at the water-air interface were interpreted as consistent with the notion that complex biomolecules could have emerged in pools of water on early Earth.
“Life” requires molecules with the ability to reproduce themselves. Proteins are usually excluded because they do not have self-replicating systems. A prime molecular example is ribose nucleic acid (RNA).3 RNA is composed of ribose, a five-carbon sugar, which is bound to a nitrogenous base, either adenine, guanine, uracil, or cytosine. The individual ribose-base units are linked by phosphodiester bonds to form polymeric strands. Phosphorus was probably available from minerals and meteorites, and could have been oxidized in solutions containing iron salts and peroxide to form the pyrophosphate essential for forming the RNA backbone.4 (Fig 1)
Base pairing refers to the hydrogen bonding that occurs between adenine-uracil and guanine-cytosine. This can produce hairpin turns within a strand and the formation of complementary strands. The polymerization of oligonucleotides is enhanced at cool temperatures. RNA can form an enzyme called RNA polymerase ribozyme that can alternatively copy itself and its complementary sequence. The authors of a recent review speculate that “molecular cooperation involving RNAs could facilitate the emergence and early evolution of life.”5
Supporting the theory of an RNA World at the time of the emergence of life, it was reported that large amounts of adenine and other bases emerged from a prebiotic atmosphere containing hydrogen cyanide and ammonia.1 The nucleobases have also been synthesized in the lab under outer space conditions using pyrimidine, a compound found in meteorites. Other prebiotic molecules have been observed in the galactic center of the Milky Way.6 Most recently, it was discovered that RNA can form on basalt lava glass, which was probably present on early Earth.7
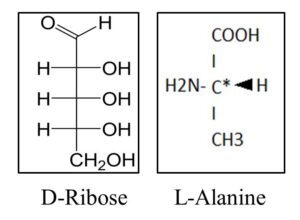
A problem with laboratory-generated molecules is that they do not exactly simulate those found in nature. The synthetic mixtures are composed of equal amounts of left- and right-handed RNA or amino-acid molecules. But in the natural world, RNA is always in the right-handed form and amino acids and proteins are left-handed (Fig 2).
Louis Pasteur, who discovered the mirror-image forms of organic molecules, thought that magnetic fields might be responsible for selecting either right- or left-handed molecules. Recent experiments support this idea.8 When ribo-aminooxazoline, an RNA precursor, is exposed to the surface of the mineral magnetite, it is sorted according to its handedness. Molecules of the same handedness form crystalline seeds that promote the binding of additional like-handed molecules.9 The ultimate outcome is the formation of homogeneous RNA building blocks that are the templates for amino acids and proteins of the opposite handedness.
Viruses, prions, and mycoplasma are small, simple entities that might have arisen on prebiotic Earth. Viruses have two basic components: a nucleic acid core and a protective protein capsid or coat. These could have been synthesized by RNA ribozymes. However, viruses are not independent life forms, and they must infect cells to reproduce. Similarly, prions are transmissible, pathologic agents that bind to prion proteins in the brain, causing them to misfold. These prion proteins form aggregates and eventually fibrils that are toxic to cells. Prions are infectious, but, like viruses, are incapable of independent existence. Mycoplasma genitalia is considered one of the smallest self-replicating organisms; its circular DNA has only 580 kb and it has no cell wall. However, it lacks enzymes to form amino acids and must acquire growth factors from its host. Thus, the identity of the original independent life-form awaits discovery.
In summary, many of the conditions existing on early Earth were favorable for the formation of the molecules required to construct living organisms. Chemicals floating in pools situated on magnetite-containing rocks could have come together to form the macromolecules needed to build the initial life-forms. They might have been supplemented by extraterrestrial compounds borne by meteorites. Although science is gradually identifying the chemical reactions that produced self-replicating molecules, it is unclear if they created living entities. It is still possible that life was cooked up by a Master Chef who arrived in a flying saucer.
References
- Miller-Urey experiment. Wikipedia. https://en.wikipedia.org/wiki/Miller-Urey_experiment.
- Griffith EC, Vaide V. In situ observation of peptide bond formation at the water-air interface. PNAS 2012; 109:15697-701.
- RNA: Relevance for prebiotic chemistry and abiogenesis. Wikipedia. https://en.wikipedia.org/wiki/RNA#Relevance_for_prebiotic_chemistry_and_abiogenesis.
- Omran A et al. Oxidative phosphorus chemistry perturbed by minerals. Life 2022;12:198. https://doi.org/10.3390/life12012098.
- Higgs PG, Lehman N. The RNA World: molecular cooperation at the origins of life. Nature Reviews/Genetics 2015;16:7-17.
- Rivilla VM et al. Molecular Precursors of the RNA World in Space: New Nitriles in the G+0.693−0.027 Molecular Cloud. Front. Astron. Space Sci. 9:876870. doi: 10.3389/fspas.2022.876870.
- Service RF. Did black volcanic rock help spark early life? Science 2022;376:1147.
- Service RF. How the molecules of life became one-handed. Science 2023;380:1094-95.
- Ritson D et al. Mimicking the surface and prebiotic chemistry of early Earth using flow chemistry. Nat Comm 2018;9. 10.1038/s41467-018-04147-2.
DAVID GREEN, MD, PhD, is a graduate of Jefferson Medical College (MD) and completed residency in internal medicine and fellowship in hematology at Jefferson and subsequently a PhD in biochemistry at Northwestern University. Over a 45-year career, he provided patient care and researched in the field of hematology. He is the author or co-author of 300 peer-reviewed scientific papers and five books.
Highlighted in Frontispiece Volume 16, Issue 1 – Winter 2024
Leave a Reply